Supramolecular approaches in transition metal catalysis
Traditionally, supramolecular catalysis focuses on mimicking the action of enzymes. Unlikeclassical chemical transformations, supramolecular catalysis relies on non-covalent interactions such as hydrogen bonding, cation-pi interaction, and hydrophobic forces to dramatically acceleratetherate of a reaction and/or allow highly selective reactions to occur.For a long time only relatively simple chemical reactions such as hydrolysis and Diels Alder reactions were successfully performed using supramolecular strategies.
We have an ongoing program on the exploration of supramolecular strategies in Transition Metal Catalysis, aiming to solve challenges in this area that are not easy to solve by traditional methods. We have introduced the use of Self-assembled bidentate ligands in transition metal catalysis, substrate orientation in transition metal catalysis and catalysis in confined spaces. These strategies have all demonstrated to provide novel tools to solve challenging issues.
a) Self-assembled ligands.
The preparation of bidentate ligands can be laborious and time-consuming, especially if the donor atoms are not identical. For the exploration of a library of ligands, a typical approach in asymmetric catalysis, this can be cumbersome and rate limiting in a typical catalyst finding process. We have demonstrated that bidentate ligands can be formed by the self-assembly of ligand components. This has been demonstrated based on metal-ligand interactions as well as hydrogen bond interactions. In fact, the effectiveness of the self-assembled ligand displayed in the figure was shown to be based on a single hydrogen bond, yet the implications for catalysis are enormous as record selectivity in asymmetric hydrogenation can be achieved. This approach is particularly valuable in combinatorial approaches. InCatT, a spin off company actively explores this approach to find new catalysts to solve issues in the chemical industry (www.incatt.eu).
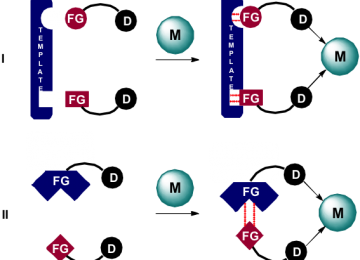
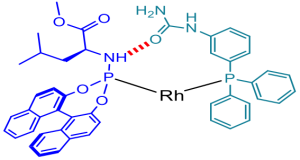
Figure. Schematic representation of supramolecular bidentates formed via a template (I), or via direct interactions between functionalized ligand building blocks (II). M = metal center, FG = functional group, D = donor center.
b) Substrate orientation effects in catalysis.
Another recently introduced strategy to achieve selectivity in transition metal catalysis is through pre-organization of the substrate with respect to the metal center. A commonly explored way to achieve pre-organization is through ditopic binding of the substrate to the metal center, in which a second functional group controls the orientation of the substrate.Recently we have explored strategies in which a substrate is boundin aditopic fashion to the metal complex, as a way to pre-organize the substrate with respect to the metal center via, usinga recognition site on the ligand. Through such supramolecular substrate pre-organization highly selective reactions can beachieved, which are not possible by classical approaches. In the figure we display two examples, DIMPhos that has been used for regioselective hydroformylation and BAIPY that has been used for regioselective C-H borylation.
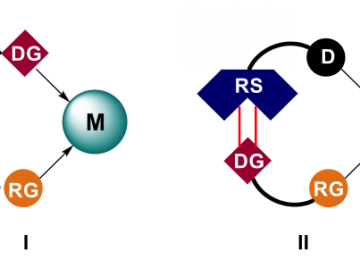
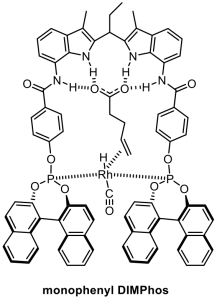
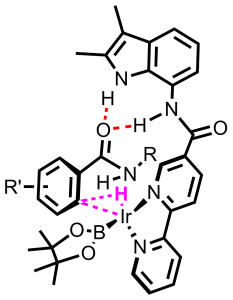
Figure. Schematic representation of substrate pre-organization via traditional approaches (I) or via supramolecular strategies(II). M = metal center, DG = directing group, RG = reactive group, RS = recognition site, D = donor center. Two examples are provided for regioselective hydroformylation and C-H activation respectively.
c) Transition metal catalysis in confined spaces.
Enzymes, Nature’s catalysts, have their active site in a well-defined pocket and as such these reactions take place in a special confined space defined by the protein cavity. Achieving similar site isolation of active transition metal complexes in confined spaces may lead to unusual reactivity and selectivity and as such has been a topic of active research in our group. We have developed over the years several strategies to put single metal complexes in cages, leading to a variety of caged catalytic systems. We have explored the performance of these caged systems in challenging reactions. Frequently observed effects are unusual selectivities, increased activities and higher stability of the catalyst.
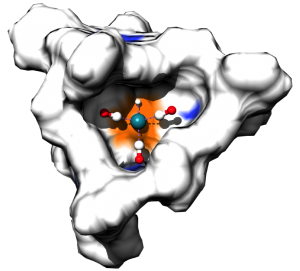
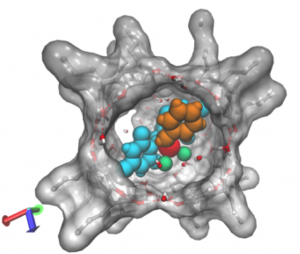
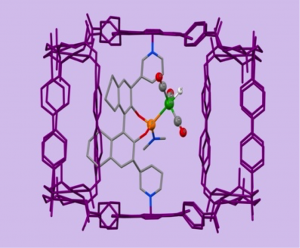
Figure. Three examples of metal complexes in cages, for hydroformylation and metathesis reactions.
Next to these single site catalysts, we have also explored the use of nanospheres to confine multiple metal sites in confined space. This leads to extremely high local concentration of metal complexes, in turn leading to special reactivity.
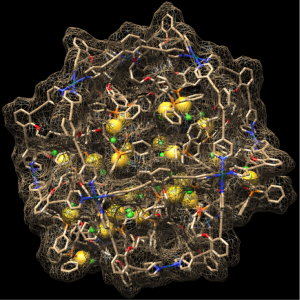
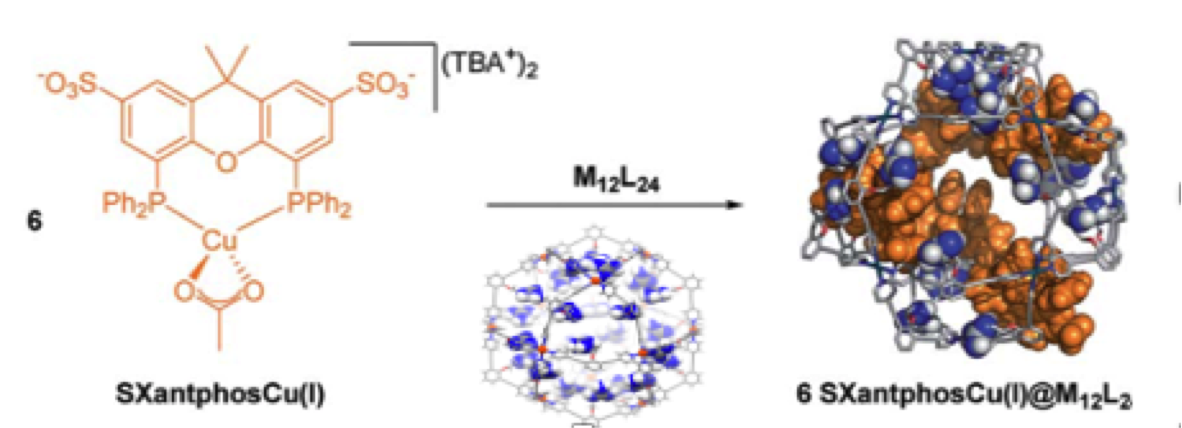
Figure. An example of a M12L24 nanosphere, loaded with 24 gold complexes, leading to a local concentration of 1.1Molar of catalyst. In the second example we non-covalently bind 6 copper catalyst in a similar nanosphere, in both examples reaction rates increase orders of magnitude because of high local concentration