Artificial photosynthesis and solar fuels
The transition to a climate-neutral society is both an urgent challenge and an opportunity to build a better future for all. As part of the European Green Deal (2020), the European Commission proposed on 4 March 2020 the first European Climate Law to enshrine the 2050 climate-neutrality target into law. This means that in 2050 the society should run on ssustainable energywith net zero emissions. In this regard solar energy is very attractive as it is abundant. In theory, one hour of incident solar power is enough to account for the yearly worldwide energy consumption. In fact, decades of research into crystalline silicon solar cellshave resulted in the production of sustainable electricity on industrial scaleand today there are available at commercially competitive pricesand power entire households. Thus 80% of the harvested solar energy is currently consumed in the form of fuel, and it is likely that fuels remain important in the future.
But there are two challenges here: 1) Electrical power, is not easily stored and transported. 2) Secondly, there is a mismatch in energy demand and solar energy supply due to the day/night cycle and the seasonal fluctuations. This means that energy storage strategies are as important as energy harvesting strategies. Energy storage and conversion requires rapid development in the coming years to allow the transition to a society that runs on sustainable energy.In this context, the production of a chemical fuel by converting solar-energy to fuel (a solar-fuel), by direct or indirect means, can become a crucial technology.Therefore, in our research group we study various aspects related to solar fuel generation, with a strong accent on the catalytic processes involved.
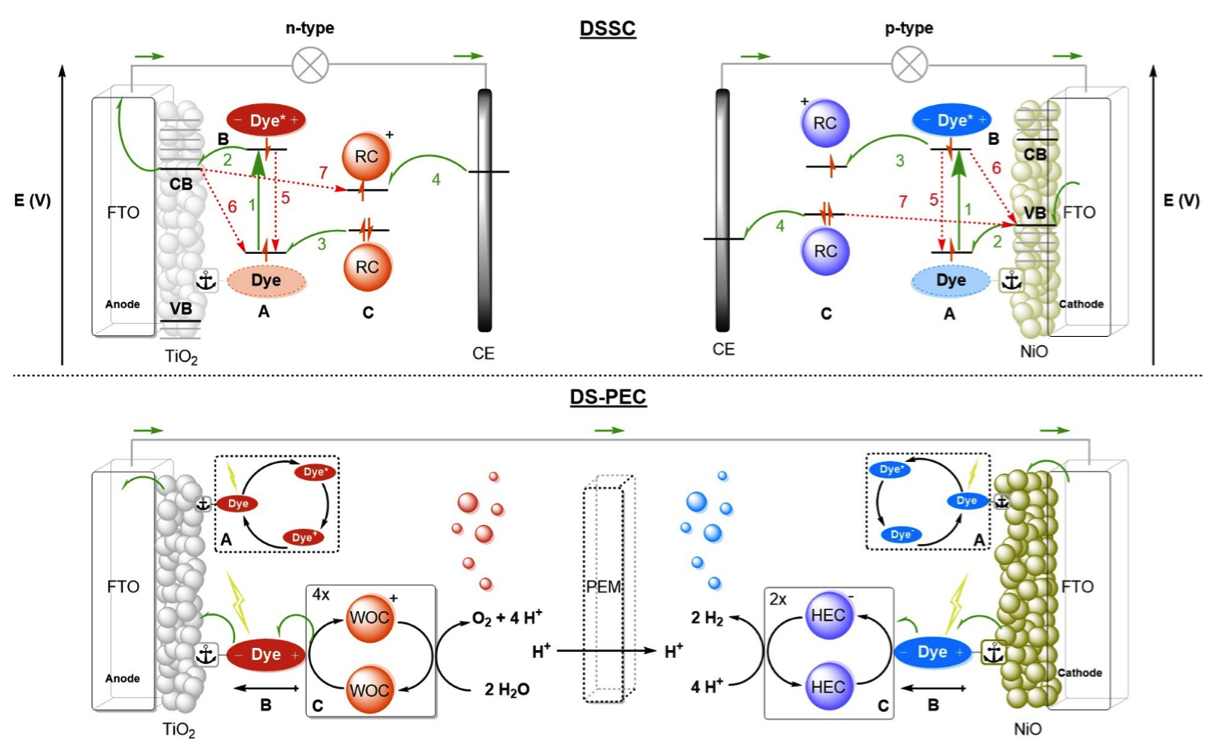
Figure. Operational mode of the DSSC (Top) and schematic diagram of a DS-PEC (Bottom)
Currently solar fuel devices based on molecular components, the so-called Dye Sensitized Photo Electrochemical Cell (DS-PEC). DSC-PECs have relatively low solar to fuel efficiencies, mostly because of competing charge recombination processes, mismatches between the catalyst properties and the device/chromophore properties.
Currently multiple projects are underway in the group where we explore strategies to improve the individual components needed in these devices as well as the devices as a whole. As a part of this research we also study new concepts in Dye sensitized solar cells (DSSC)as the fundamental basis of these devices is very similar.
Some typical applications of the approaches that we develop are in the improvement of water oxidation catalysts. For example, by combining concepts of pre-organization in nanospheres we have been successful in encapsulating different hydrogenase mimics in a variety of cages. This leads to more favourable proton reduction catalysis. In addition to that, to prevent charge recombination we have developed molecular machinery that arranges directional charge migration, leading to reduced charge recombination in DSSCs, and as a result in higher solar to current efficiencies.

Figure. Sulfonated water oxidation catalystsenclosed in a M12L24 supramolecular sphereto increase reaction rates by factor 140.

Figure. A hydrogenase mimic in a molecular cage that leads to proton reduction catalysis at more favorable potential.

Figure. Supramolecular interactions are implemented in p-type DSSC using the “blue box” as redox shuttle. The PN dye is heterogenized onto NiO, and binds the ring prior to excitation. As soon as the ring is reduced, it loses affinity for the chromophore, dethreads and moves away while carrying the charge.